Mechanochemistry
Mechanical Characterization and Mechanochemistry
Mechanical-electrochemical phenomena exhibit many interesting multidirectional couplings in ion-exchange polymers due to their intrinsic material physio-chemical states and responses to environmental stressors. Understanding the origins of mechanochemistry and probing the chemical-mechanical interactions would have a significant impact on technologies that exploit these phenomena, from solid-polymer electrolytes to functional composites used in electrochemical energy devices.
Chemical-Mechanical Stability of Functional Polymers
Our research focuses on the characterization of the chemical and mechanical stressors as well as an understanding of the interrogation of their synergistic effects affecting material function and lifetime for fuel cells and electrochemical devices operating based on similar principles, such as alkaline fuel cells and water-splitting electrolyzers. We aim to identify the stressors in these devices and develop suitable characterization procedures that could help probe the membrane's stability under realistic conditions.
Mechanical Characterization of Electrolyzer Membranes
Proton-exchange membrane (PEM) water electrolyzers (PEMWEs) are a promising energy technology for electrochemical generation of low-cost hydrogen by splitting water using electricity. Key is to produce affordable electrolytic hydrogen without sacrificing durability or efficiency. This is the goal of the DOE-funded H2NEW consortium, which aims to enable affordable, reliable, and efficient electrolyzers to achieve low‑cost hydrogen.
Our research focuses on enhancing the durability of electrolyzer membranes by providing a scientific understanding of the underlying factors controlling membrane stability during device operation. For electrolyzers to be commercially feasible, PEMs must perform over long lifetimes in liquid environments under compression while maintaining mechanical stability.
PEMWEs use a solid-electrolyte polymers membrane as the separator which functions in a hydrated environment. Such a hydrated environment, while inherent for operation and proton conductivity, undermines PEM stability. Mechanical stability of PEMs is commonly characterized in tension, which is not applicable to electrolyzers, wherein PEMs could undergo high pressures. In this project, a compression creep procedure is developed using a custom-designed setup to monitor the creep response of hydrated PEMs. Our recent results show that PEMs exhibit creep under compression, with a dependence on the pressure and hydration, which signifies to the importance of mechanical characterization under stressors relevant to device operation.
Chemical-Mechanical Durability of Fuel-Cell Membranes
Polymer-electrolyte fuel cells (PEFCs) are energy-conversion devices with great potential to supply electrical power for transportation and stationary applications. PEFCs are required to perform over long operational times without the failure of cell components, in particular, the polymer-electrolyte membrane.
The role of the membrane is to provide a conductive pathway for protons (anode to cathode) while blocking the crossover of reactants, such as H2 and O2. In PEFCs, the crossover of these species leads to the formation of hydroxide radicals which attack the membrane's chemical structure. The chemical decomposition of the membrane during operation is accompanied by the physical defects that arise due to mechanical stresses caused by cell assembly and the operating environment, in particular, swelling of the hydrophilic membrane in the presence of water. The combination of these chemical & mechanical stressors enhances the defect formation and crossover, which not only reduces power loss but also acts to further accelerate the intensity of the stressors. Due to these complex interactions between the chemical and mechanical failure modes in a PEFC, it is imperative to understand the relationship between different failure modes, isolate their individual roles, and the dominating mechanisms that control the material lifetime.
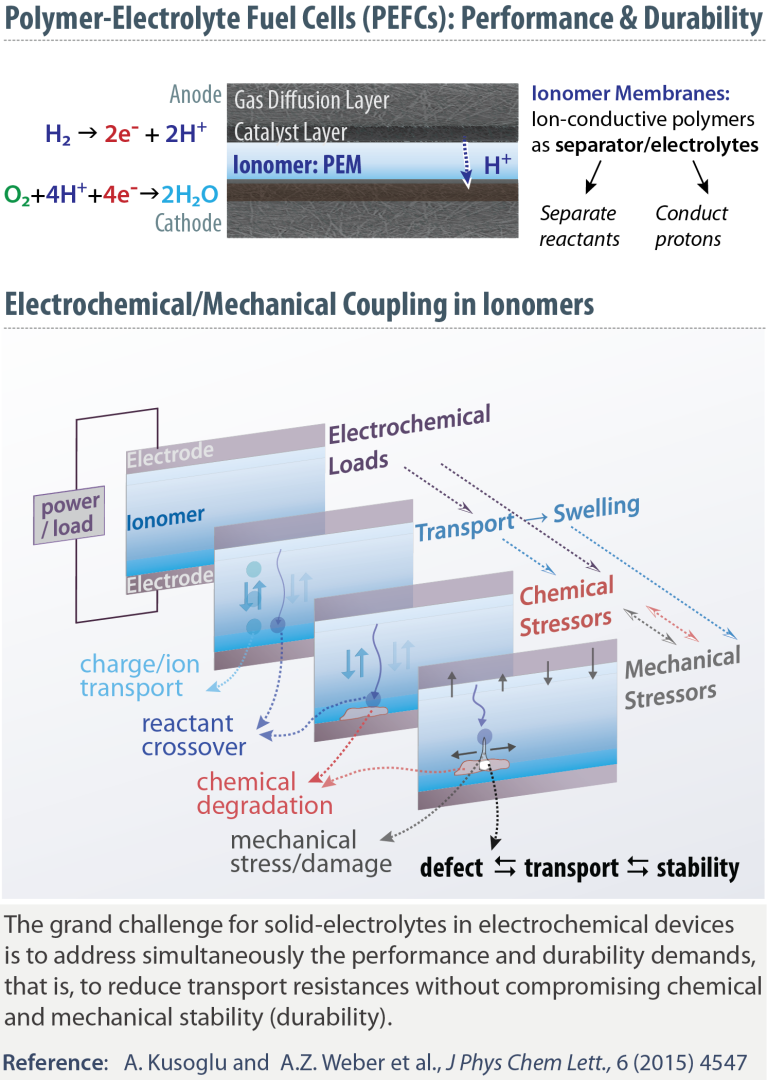