Ionomers
Ionomers: Ion-containing Polymers
Ionomers are ion-containing polymers where the ionic groups are covalently bonded to the polymers’ backbone. These ionic groups are neutralized by mobile ions of opposite charge (counter-ions), which gives an ionomer its ion-conductive properties, a critical functionality for transporting ions in solid-electrolyte membranes. Our research involves structural characterization and modeling of transport and mechanical properties of ion-conductive polymers and composites to establish their structure-function-durability relationships and develop design guidelines for electrochemical energy applications.
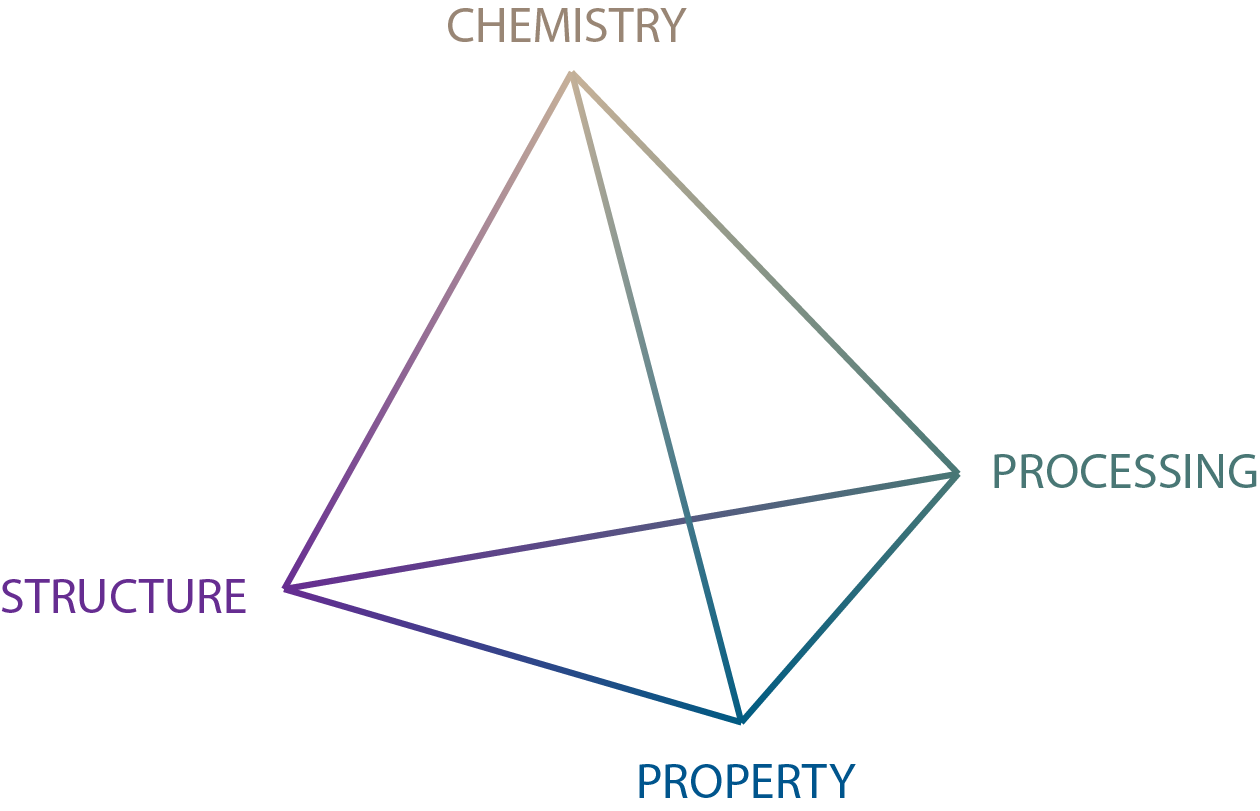
Most of the current understanding of ionomers is derived from decades-long research on Perfluorinated sulfonic acid (PFSA), the most commonly used proton-exchange membrane (PEM) in fuel cells owing to their high conductivity and chemical-mechanical durability. PFSAs have served as the benchmark ionomer, yet the knowledge gained from their integration and durability studies could guide the design and development of their derivatives and alternative membranes tailored for specific applications, from fuel cells to electrolysis by accounting for their operating conditions and durability stressors. The overarching goal is to elucidate and establish the relationships among chemistry, structure, processing effects, and properties and investigate how they collectively govern the ionomer function and response in devices.
Proton and Cation-Exchange Ionomer Membranes
A common use of solid-electrolyte membrane in energy technologies is proton-conduction, in which case the ionomer function as a proton-exchange membrane (PEM). The most widely used ionomer for PEM, especially in fuel-cells, is Perfluorosulfonic-acid (PFSA), due to its remarkable proton transport properties and chemical-mechanical stability. While the high proton conductivity of PFSA is attributed to its hydrophilic sulfonic-acid groups, and their hydration with water, the stability of this ionomer is imparted by its hyrophobic polytetrafluoroethylene (PTFE) backbone. Any factor that improves transport properties of an ionomer tend to undermine its stability, thereby creating a transport/stability trade-off. An active area of research is to understand how ionomer chemistry and environmental factors control the interplay between the transport and stability of the ionomers and to develop high-performance and durable materials for electrochemical energy devices.
PFSA ionomers are widely used as solid-electrolyte ion-exchange membranes in electrochemical devices, wherein their properties are impacted by the interactions among the anionic sulfonate groups, mobile counter-ions (cations), and hydration levels. Cation-form and humidity collectively affect the structure/transport-property relationship, yet their interplay is coupled through the water-cation interactions and phase-separated nanomorphology. In a recent paper, we studied water uptake and conductivity of cation-exchanged PFSA in both vapor and liquid water, and then correlated them with changes in mechanical properties and nanostructure (hydrophilic-domain spacing and phase-separation) to establish transport/stability relationships.
With increasing cation size (radius) and valence, the storage modulus increases, while the water uptake and conductivity decreases yielding a universal relationship indicative of a transport/stability tradeoff. The extent to which the cations impact the transport properties depends on the water content: at low hydration levels the controlling factor is the cation (and its interaction with the sulfonate sites), and with increasing hydration and in liquid water the dominant factor becomes water volume fraction, although it is also controlled by the cations. In liquid water, the conductivity scales with the water volume fraction, regardless of the cation type and valence. However, as the degree of hydration is reduced the conductivity data begins to deviate from this trend, where conductivity depends more on the cation’s interaction with the sulfonate group, and its dissociation, more so than the water content. Similarly, the decrease in hydrophilic domain spacing of PFSA exchanged with larger cations scales with cation radius at low water contents, but with Lewis acid strength (LAS) at higher hydration levels. Overall, it was found that, it is the water per charge, rather than the water per sulfonate group, that appears to govern the structure/transport relationship.
Composite Ionomer Membranes
Anion-Exchange Ionomer Membranes
Alkaline anion-conducting polymer-based CO2 electrolysis and water electrolysis are among two emerging renewable energy conversion technologies. Their system design and integration offer promise of lower capital cost due to utilization of low-cost catalysts, in contrast to platinum group metal catalysts required for cation-conducting polymer-based devices. However, a critical component, the polymer electrolyte membrane, remains an obstacle hampering system performance and durability. In a recent study by our group, commercially-available membranes with and without PTFE-reinforcement were investigated to understand previously unreported origins of improved device performance when compared to alternative membrane chemistries. We report critical membrane properties, such as morphology, thermal stability, as well as temperature-, hydration-, and counter-ion dependent ion conductivity. Moveover, the changes in uptake and conductivity of membranes in supporting electrolytes of K2CO3 and KOH investigated as a function of their concentration. The presence of reinforcement and supporting electrolyte type alter the membrane's transport functionality, which could help guide device design for improved performance. The obtained results not only show how AEM change with operating environment for CO2 and water electrolysis applications, but also provide understanding for internal and external factors controlling anion-exhcnage membrane functionality in electrochemical devices.
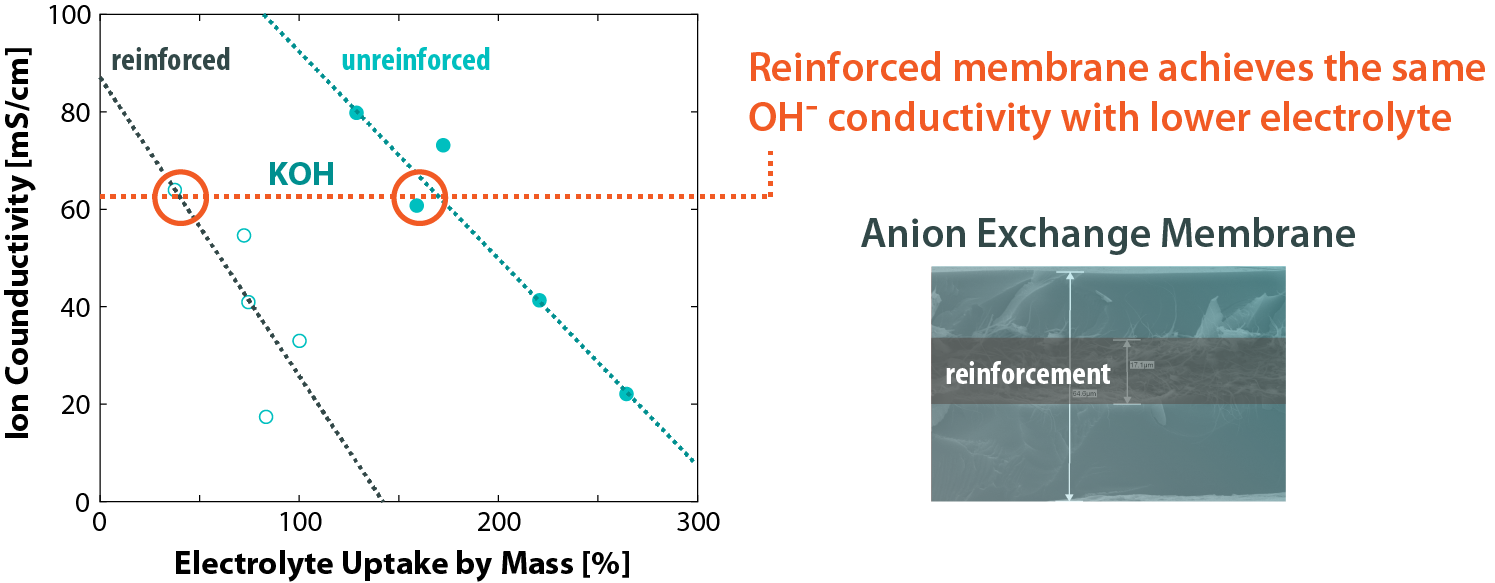